you might want to spend some time learning the basics, so read the links below,
the most common mistake made by many people is that they fail to look at an engine as an interconnected group of component sub systems and they don,t realize that changes to a single component, no mater how much potential that component has is not going to allow that component or change in the potential to be realized until all the matched and supporting systems have similar potential.
EXAMPLE ,
the heads may be capable of flowing (x) on a stock engine but with careful selection of a cam with the correct duration and lift, and with a tuned header, and matching valve train mods along with some port and bowl clean-up the resulting improvements can be significantly more impressive.
http://garage.grumpysperformance.com/index.php?threads/is-backpressure-hurting-your-combo.495/
http://garage.grumpysperformance.co...alves-and-polishing-combustion-chambers.2630/
http://garage.grumpysperformance.co...e-springs-and-setting-up-the-valve-train.181/
http://garage.grumpysperformance.co...lsa-effects-your-compression-torque-dcr.1070/
http://garage.grumpysperformance.com/index.php?threads/more-port-flow-related-info.322/
http://garage.grumpysperformance.co...2-bbc-rebuild-but-think-things-through.13604/
http://garage.grumpysperformance.co...t-intake-on-oval-port-heads.13146/#post-69975
http://garage.grumpysperformance.com/index.php?threads/calculating-ideal-port-size.624/
http://garage.grumpysperformance.com/index.php?threads/carb-intake-manifold-engine-flow-rates.10194/
http://documents.holley.com/techlibrary_selecting_a_carburetor.pdf
http://www.wallaceracing.com/intakecfm.php
Volumetric Efficiency
Actual CFM
= Volumetric Efficiency
Theoretical CFM
viewtopic.php?f=55&t=2994&p=32443&hilit=carb+restrictive#p32443
http://www.hipermath.com/engines/carburetor_cfm
http://www.enginebasics.com/Advanced%20 ... ooler.html
http://www.summitracing.com/expertadvic ... Calculator
http://gottafishcarburetors.com/CFM Formula.html
http://wallaceracing.com/intakecfm.php
Volumetic Efficiency or VE, I will be using from this point, varies depending on temperature and pressure.
From that, we know a normally aspirited engine will have VE of 100% or less. And force inductioned engine will have VE of 100% or more.
The actual calculation of VE is done by ECU using measured amount of intake air, with Mass Air Sensor measuring at intake pipe or Speed Density measuring inside the intake manifold (close to intake port of the engine).
Theoretical CFM
theoretical cfm = rpm x displacement / 3456
Engine Flow Demand
Engine Flow= (engine displacement) X (volumetric efficiency) X (engine speed) X (manifold pressure)
You can see the key to increase engine flow is to increase engine VE (volumetric efficiency). Reduce intake charge temperature is the easiest way to help increase engine VE. This is where air/air, air/fluid intercooler and water injection come into play.
Engine Flow Measurement
Most engine control systems in production today utilize either speed-density sensors or air-mass sensors to measure engine air flow. Speed-density is very popular because of its low cost and high reliability. Speed-density systems typically calculate engine flow rate based on engine speed, intake manifold pressure and temperature. Some systems use barometric pressure sensors and inlet air temperature sensors to improve flow calculation accuracy for varying ambient conditions. Fig. 1 illustrates a typical engine with both speed-density sensors and an air-mass sensor.
read these related links
http://tunertools.com/articles/volumetr ... ciency.asp
http://www.ajdesigner.com/phpengine/eng ... ciency.php
http://www.installuniversity.com/instal ... 012000.htm
http://www.epi-eng.com/piston_engine_te ... ciency.htm
http://www.ztechz.net/id2.html
an engines Torque peak is almost always very closely related to the point in the rpm curve where the most effective/efficient cylinder fill, cylinder fill is related to both intake port cross sectional area and exhaust scavenging,efficiency, and is limited by port stall, and cam duration in relation to displacement, compression and valve train stability, ...all factors are easily calculated
links below
useful RELATED INFO you might want to read
http://www.wallaceracing.com/runnertorquecalc.php
http://www.pontiacracing.net/js_header_length1.htm
http://www.superchevy.com/how-to/en...-0902-chevy-engine-port-variations-measuring/
http://garage.grumpysperformance.com/index.php?threads/port-speeds-and-area.333/
http://garage.grumpysperformance.com/index.php?threads/calculating-ideal-port-size.624/
http://garage.grumpysperformance.com/index.php?threads/sellecting-cylinder-heads.796/
http://garage.grumpysperformance.co...ting-a-port-size-and-intake-for-nitrous.4930/
http://garage.grumpysperformance.com/index.php?threads/semi-fool-proof-cam-sellection.82/
http://garage.grumpysperformance.co...velocity-heads-tuned-intake-turbulence.12998/
http://garage.grumpysperformance.com/index.php?threads/calculating-header-design.185/
the most common mistake made by many people is that they fail to look at an engine as an interconnected group of component sub systems and they don,t realize that changes to a single component, no mater how much potential that component has is not going to allow that component or change in the potential to be realized until all the matched and supporting systems have similar potential.
EXAMPLE ,
the heads may be capable of flowing (x) on a stock engine but with careful selection of a cam with the correct duration and lift, and with a tuned header, and matching valve train mods along with some port and bowl clean-up the resulting improvements can be significantly more impressive.
http://garage.grumpysperformance.com/index.php?threads/is-backpressure-hurting-your-combo.495/
http://garage.grumpysperformance.co...alves-and-polishing-combustion-chambers.2630/
http://garage.grumpysperformance.co...e-springs-and-setting-up-the-valve-train.181/
http://garage.grumpysperformance.co...lsa-effects-your-compression-torque-dcr.1070/
http://garage.grumpysperformance.com/index.php?threads/more-port-flow-related-info.322/
http://garage.grumpysperformance.co...2-bbc-rebuild-but-think-things-through.13604/
http://garage.grumpysperformance.co...t-intake-on-oval-port-heads.13146/#post-69975
http://garage.grumpysperformance.com/index.php?threads/calculating-ideal-port-size.624/
http://garage.grumpysperformance.com/index.php?threads/carb-intake-manifold-engine-flow-rates.10194/
http://documents.holley.com/techlibrary_selecting_a_carburetor.pdf
http://www.wallaceracing.com/intakecfm.php
Volumetric Efficiency
Actual CFM
= Volumetric Efficiency
Theoretical CFM
viewtopic.php?f=55&t=2994&p=32443&hilit=carb+restrictive#p32443
http://www.hipermath.com/engines/carburetor_cfm
http://www.enginebasics.com/Advanced%20 ... ooler.html
http://www.summitracing.com/expertadvic ... Calculator
http://gottafishcarburetors.com/CFM Formula.html
http://wallaceracing.com/intakecfm.php
Volumetic Efficiency or VE, I will be using from this point, varies depending on temperature and pressure.
From that, we know a normally aspirited engine will have VE of 100% or less. And force inductioned engine will have VE of 100% or more.
The actual calculation of VE is done by ECU using measured amount of intake air, with Mass Air Sensor measuring at intake pipe or Speed Density measuring inside the intake manifold (close to intake port of the engine).
Theoretical CFM
theoretical cfm = rpm x displacement / 3456
Engine Flow Demand
Engine Flow= (engine displacement) X (volumetric efficiency) X (engine speed) X (manifold pressure)
You can see the key to increase engine flow is to increase engine VE (volumetric efficiency). Reduce intake charge temperature is the easiest way to help increase engine VE. This is where air/air, air/fluid intercooler and water injection come into play.
Engine Flow Measurement
Most engine control systems in production today utilize either speed-density sensors or air-mass sensors to measure engine air flow. Speed-density is very popular because of its low cost and high reliability. Speed-density systems typically calculate engine flow rate based on engine speed, intake manifold pressure and temperature. Some systems use barometric pressure sensors and inlet air temperature sensors to improve flow calculation accuracy for varying ambient conditions. Fig. 1 illustrates a typical engine with both speed-density sensors and an air-mass sensor.
read these related links
http://tunertools.com/articles/volumetr ... ciency.asp
http://www.ajdesigner.com/phpengine/eng ... ciency.php
http://www.installuniversity.com/instal ... 012000.htm
http://www.epi-eng.com/piston_engine_te ... ciency.htm
http://www.ztechz.net/id2.html
an engines Torque peak is almost always very closely related to the point in the rpm curve where the most effective/efficient cylinder fill, cylinder fill is related to both intake port cross sectional area and exhaust scavenging,efficiency, and is limited by port stall, and cam duration in relation to displacement, compression and valve train stability, ...all factors are easily calculated
links below
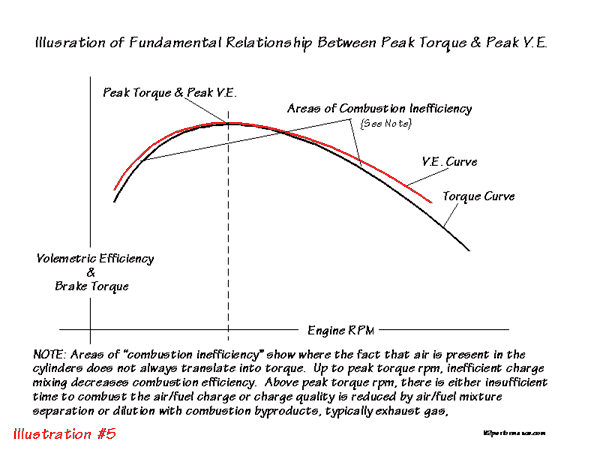
useful RELATED INFO you might want to read
http://www.wallaceracing.com/runnertorquecalc.php
http://www.pontiacracing.net/js_header_length1.htm
http://www.superchevy.com/how-to/en...-0902-chevy-engine-port-variations-measuring/
http://garage.grumpysperformance.com/index.php?threads/port-speeds-and-area.333/
http://garage.grumpysperformance.com/index.php?threads/calculating-ideal-port-size.624/
http://garage.grumpysperformance.com/index.php?threads/sellecting-cylinder-heads.796/
http://garage.grumpysperformance.co...ting-a-port-size-and-intake-for-nitrous.4930/
http://garage.grumpysperformance.com/index.php?threads/semi-fool-proof-cam-sellection.82/
http://garage.grumpysperformance.co...velocity-heads-tuned-intake-turbulence.12998/
http://garage.grumpysperformance.com/index.php?threads/calculating-header-design.185/
Last edited by a moderator: