quench area:
http://www.chevyhiperformance.com/tech/ ... index.html
http://www.chevyhiperformance.com/techa ... index.html
http://www.rbracing-rsr.com/squishcalc1.html
http://www.klotzlube.com/techsheet.asp?ID=87
[color=#008000[b]]read thru these threads also[/b][/color]
www.enginelabs.com
viewtopic.php?f=52&t=4081&hilit=tumble
detonation and rapid heat increases can ruin piston ring seal to the bore wall
Mahle pistons are designed for specific applications with the alloy that is best suited for that particular application.
but keep in mind the better versions of forged pistons tend to have the advantage in both strength and heat tolerances
......................Piston Alloy Comparison
4032...................................................... 2618
High silicon............................................No silicon
Low expansion........................................expansion
Tighter piston-to-wall clearance................More Piston-to-wall clearance needed
Quiet Operation......................................Noise when cold
Less ductile............................................More ductile
More stable & consistent.........................Higher resistance to detonation
Longer life cycles....................................Shorter life cycles
Harder...................................................Softer
A zone in the combustion chamber where the flat area of the piston at top dead center is very close to the matching flat area on the combustion chamber in the cylinder head. Because the piston and cylinder head is significantly cooler than the as yet unburned part of the fuel-air mixture (i.e., end gas), they pull the heat from the fuel/air mix trapped between them at tdc , . Because the end gas is now cooler, detonation is quenched or reduced. this mass of fuel/air mix is thrown into the center of the combustion process much like a raw egg would be rapidly exiting from the area between a table top and a rubber mallet if you rapidly smacked down on it, but unlike the egg the combustion chamber contains and directs the splash in a known direction due to its design and clearances However, the process does form unburned hydrocarbons. the quench area is between the piston at top dead center and the flat surface of the cylinder head it consists of the distance between the two, limited to the compressed head gasket thickness and the distance below or above the block surface the piston extends,the OBJECT of including an EFFECTIVE quench area is to force a jet of rapidly moving fuel/air mix to shoot out into the combustion chamber, resulting in a far faster and more efficient burn in the combustion chamber, clearance of less than about .036 results in the piston hitting the heads in some applications, distances over about .044 results in a far less effective jet of fuel/air mix and potential for detonation to occur in the quench area, remember it only works if the head and piston get so close that theres a boundary layer of semi cooled air that's so tight it can,t ignite.
quench acts a bit like throwing a cup of gasoline, hard and fast into a camp fire.......it results in a huge increase in the burn rate compared to pouring the gas slowly into a fire
keep in mind ONLY pressure resulting from the fuel/air mix occurring AFTER TDC adds power or torque, any pressure or fuel burnt during the compression stroke tends to reduce power
example
if the piston was .020 down the bore at TDC and the compressed head gasket was .024 the quench distance would be .044, but in reality at high rpms its a bit tighter because pistons and rods stretch at high rpm levels just a bit.
try to keep the QUENCH in the .038-.042 for the most effective results, naturally milling the heads has no effect on quench, but milling the block does, simply because while milling the heads raises compression, by making the combustion chamber smaller in most engines it doesn,t change the quench distance, milling or decking the block changes the distance between the piston and head surface so that does alter quench.
SQUISH
An area in the combustion chamber of some engines where the piston squishes or squeezes part of the fuel-air mixture at the end of the compression stroke. As the piston approaches top dead center, the mixture is pushed out of the squish area and this promotes turbulence, further mixing of the fuel-air mixture and more efficient combustion
run less than about .037 thousands and at high rpm levels the pistons might hit the cylinder heads, run more than about .044 thousands the QUENCH effect of forcing the fuel air mix to the center of the cylinder from the cylinders edge area looses both speed and effectiveness, remember the quench area must be so tight that virtually all the fuel/air mix is forced (squished) into the center area and none is allowed to burn until its squirted into the burn area increasing turbulence and burn efficiency
in theory the much better quench, combined with the shorter more compact area the flame front needs to cover and the far higher turbulence combine to allow more of the pressure to build AFTER the crank passes TDC on the end of compression and beginning of the power stroke
its mostly an advantage in that you get a more even and FASTER burn in the cylinder and less chance of detonation, simply because both the lower time and faster pressure curves favor the ignition flame front vs detonation
look, it takes approximately 40 thousands of a second for the flame from the ignition to cross a 4.25" bore,at low rpms and still takes about 15 milliseconds at high RPM due to the much faster movement of the compressed fuel air mix in the cylinders, lets look at what that means
if the Chevy plug is located 4/5ths of the way to one side that's a time of about 32 thousands for the pressure to build as the flame travels 3.4" in the Chevy but in a compact combustion chamber it could only take the cylinder flame front less than 10-20 thousands of a second to travel across the combustion chamber for a complete burn at low rpms, this of course speeds up as the swirl and turbulence increase with increased engine RPMs but the ratios stay similar. this results in more usable energy WORKING on the piston AFTER IT PASSES TOP DEAD CENTER ON THE POWER STROKE. BUT MODERN WEDGE combustion chambers use increased QUENCH to speed the flame front and lower the burn time combined with a smaller combustion chambers.
the difference may be easier to grasp if you think of the quench area as a significant part of the total combustion chamber volume,that's forcing its potential fuel/air mix into the central combustion chamber as a jet of highly compressed F/A mix, like the difference between lighting a cup of gasoline by simply placing it next to a camp fire vs throwing it violently into a camp fire
naturally you need to verify the piston to deck height
the quench (squish) at .035 would be unlikely to be a problem from the octane being to low, angle, but rather a huge potential for the piston too head contact as the rpms build and the rods stretch on the exhaust strokes where there's little compression to slow the rod stretch as they play (crack the whip) at high rpms and if you don,t think rods and pistons expand under high rpm and high heat then you've probably never seen the marks the heads quench areas leaves on heads/pistons at quench distances lower than about .037 which Ive found its best to exceed and stay in the .038-.042 range.
yes its entirely possible to run a .035 quench, but you better be running top quality components and measure very carefully.
get out a .035 feeler gauge and look at it on edge then rock the piston in the bore slightly and you think about it a bit.
http://em-ntserver.unl.edu/Mechanics-Pages/Luke-schreier/unzip/Tension and Compression in Connecting Rods VI.htm
the quench/squish area is generally located opposite the spark plug location side of the bore, and there's generally a matching flat area on the combustion chamber
here the dome and spark plug side is on the right and the flat quench/squish is on the left
here is a cylinder head
the spark plug and dome goes to the exhaust side,(lower edge) the quench squish area is the matching flat area towards the intake on these sbc heads (upper edge in this picture)
the idea, is that as the piston almost impacts the head the two flat areas force the cylinder volume trapped between the flat areas violently toward the spark plug , this speeds the burn rate and tends to limit detonation
think of clapping your hands together violently with a raw egg in your palm, that raw egg will be forced away from the impending impact and squeezed out to spray to the sides, the cylinders volume get sprayed the same way but limited by the bore and chamber in only towards the spark plug direction, thus a high velocity mist of gas and air gets thrown into the area of ignition just as the burn starts, speeding the burn rate and lowering the distance the flame must cross
look at this picture, in a real engine the distance is very tight unlike the diagram and a good percentage of the cylinder volume is compressed and forced into the dome/spark plug area
generally you measure the piston to block/deck height and then add the compressed head gasket thickness to the deck height distance to find the heads distance from the piston, but cross check with
a dial indicator on a deck bridge
http://store.summitracing.com/partdetail.asp?autofilter=1&part=PRO-66797&N=700+115&autoview=sku
and a dial indicator
http://store.summitracing.com/partdetail.asp?autofilter=1&part=PRO-66962&N=700+-111345+115&autoview=sku
and re- check with plasti-Gage during the pre- assembly
http://www.kb-silvolite.com/article.php ... ad&A_id=56
http://www.kb-silvolite.com/article.php ... ad&A_id=36
http://www.cswnet.com/~carother/compression_ratio.htm
http://www.kb-silvolite.com/test/articl ... ad&A_id=35
and before someone points out that plasti-Gage won,t measure .038-.042, yeah! your correct by itself it wont but Ive cut dime size end tabs from a cheap feeler gauge
http://www.mytoolstore.com/kd/kdfeel01.html
next time your looking
ASK FOR A TAPPET GAUGE
for some reason most auto parts stores don,t seem to have feeler gauges but do have (TAPPET GAUGES)
http://www.jcwhitney.com/jcwhitney/prod ... map=27994G
and I usually use a .035 tab with a cross of plasti-gauge on top set on the quench area, its easily placed and removed and measured after spinning the engine over and there's no chance of damage to the piston or head,
lots of guys just use soft solder and a machinists mic., and it works fine in most cases, the potential problem with that is that solder forces the piston to compress the solder and it takes a good deal MORE force to compress solder than a thin thread of plastic, when the rods in compression you'll get a similar clearance, but when the rods whipping the piston around on the exhaust stroke under TENSION that quench distance tends to be closer to the head as the rod,piston and clearances tend to stretch out due to heat and inertial loads due to centrifugal forces, you need to be sure the piston wont hit the head on both the exhaust and compression strokes and holding the piston down, away from the head crushing solder tends to give a slightly wider clearance because it tends to rock the piston in its bore, firmly away from the quench clearance on its piston pin.
yeah! Ive done it both ways and the difference is minor but there is still a difference of a couple thousands, probably not significant in most cases but get that clearance real tight and it might be critical info to know
ID suggest trying for a MINIMUM of .038-.044 on a 496 BBC for quench as the long stroke bbc, or any BBC run over about 6000rpm with a 4" or longer stroke) tends to allow the quench clearance distance to tighten up a bit at high rpms, as parts stretch under stress/inertial loads
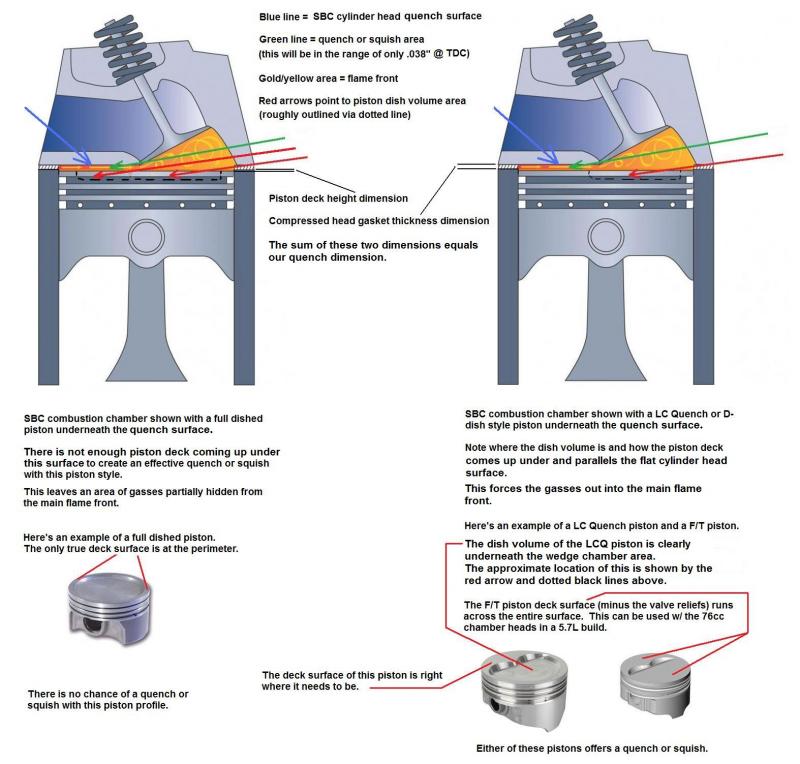
http://www.chevyhiperformance.com/tech/ ... index.html
http://www.chevyhiperformance.com/techa ... index.html
http://www.rbracing-rsr.com/squishcalc1.html
http://www.klotzlube.com/techsheet.asp?ID=87
[color=#008000[b]]read thru these threads also[/b][/color]
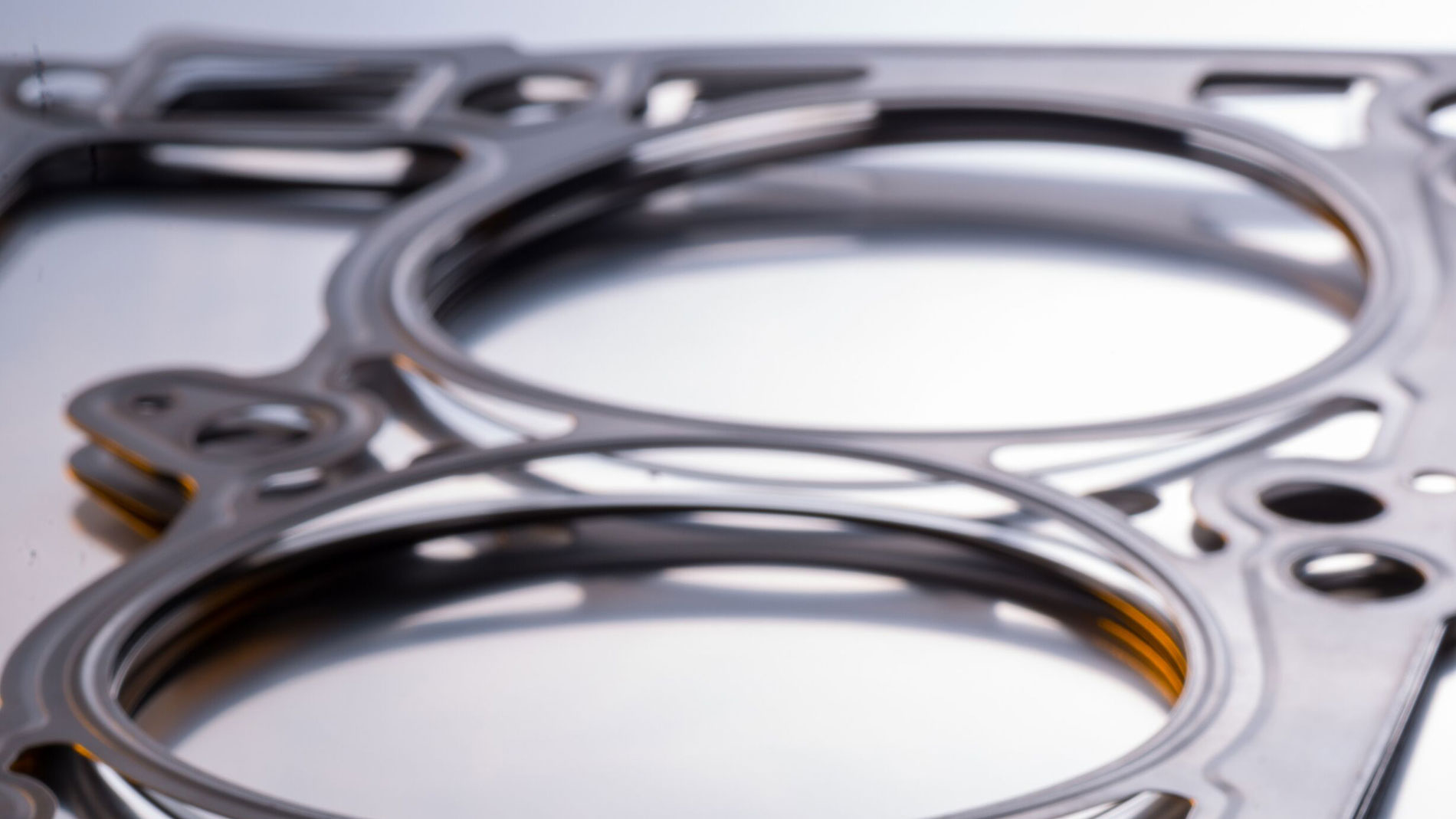
Quench: Unlocking Performance Squished Between the Piston and Head
Quench is the clearance between the piston and cylinder head at TDC. It can affect engine effeciency and performance, if you truly understand it.
viewtopic.php?f=52&t=4081&hilit=tumble
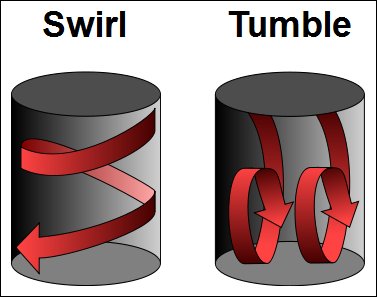
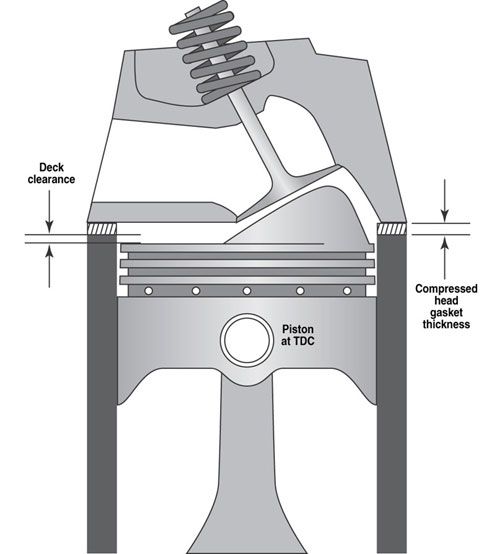
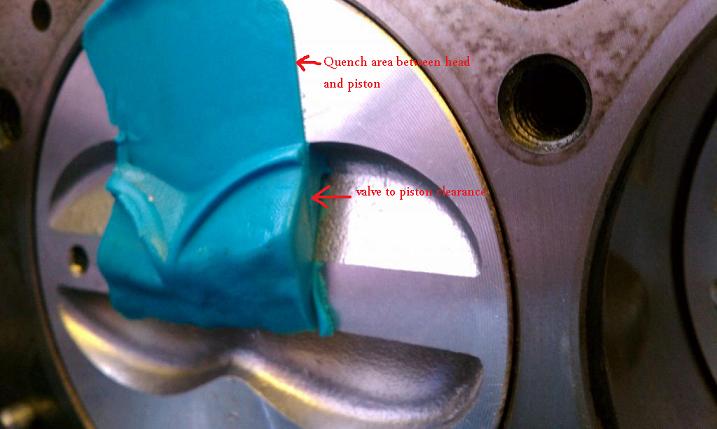
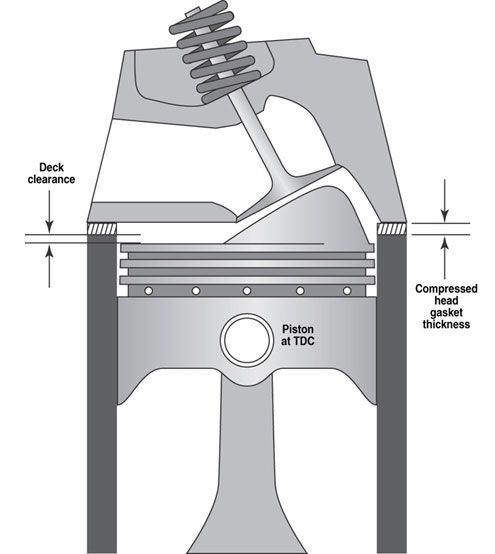
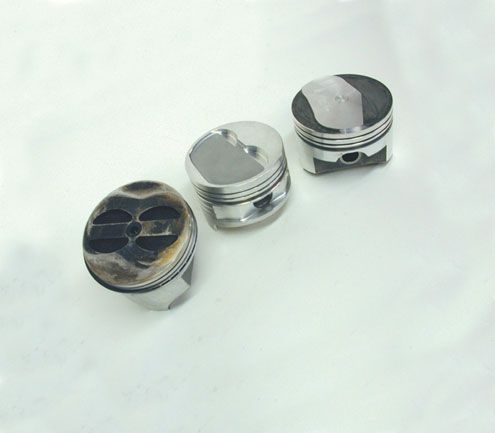
detonation and rapid heat increases can ruin piston ring seal to the bore wall
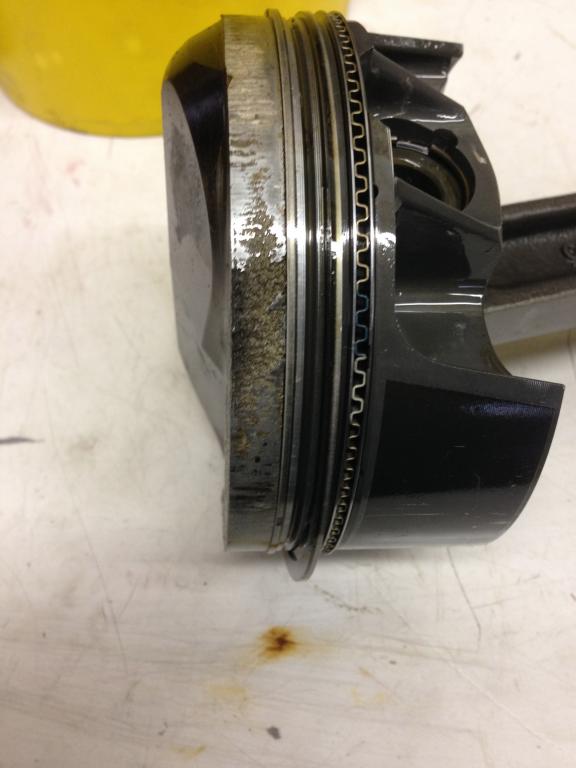
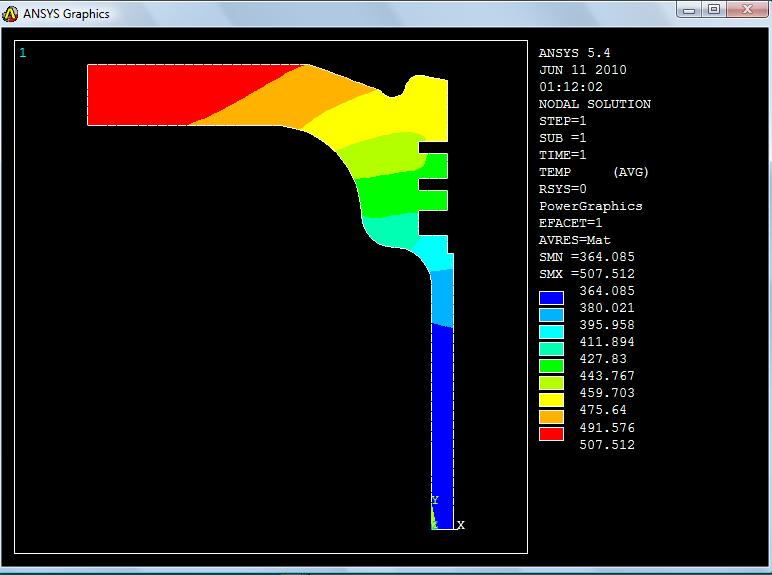
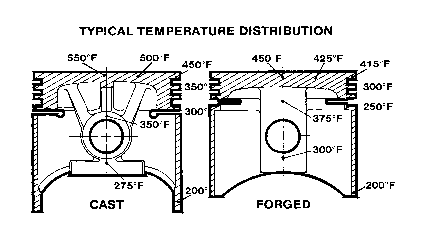
Mahle pistons are designed for specific applications with the alloy that is best suited for that particular application.
but keep in mind the better versions of forged pistons tend to have the advantage in both strength and heat tolerances
......................Piston Alloy Comparison
4032...................................................... 2618
High silicon............................................No silicon
Low expansion........................................expansion
Tighter piston-to-wall clearance................More Piston-to-wall clearance needed
Quiet Operation......................................Noise when cold
Less ductile............................................More ductile
More stable & consistent.........................Higher resistance to detonation
Longer life cycles....................................Shorter life cycles
Harder...................................................Softer
A zone in the combustion chamber where the flat area of the piston at top dead center is very close to the matching flat area on the combustion chamber in the cylinder head. Because the piston and cylinder head is significantly cooler than the as yet unburned part of the fuel-air mixture (i.e., end gas), they pull the heat from the fuel/air mix trapped between them at tdc , . Because the end gas is now cooler, detonation is quenched or reduced. this mass of fuel/air mix is thrown into the center of the combustion process much like a raw egg would be rapidly exiting from the area between a table top and a rubber mallet if you rapidly smacked down on it, but unlike the egg the combustion chamber contains and directs the splash in a known direction due to its design and clearances However, the process does form unburned hydrocarbons. the quench area is between the piston at top dead center and the flat surface of the cylinder head it consists of the distance between the two, limited to the compressed head gasket thickness and the distance below or above the block surface the piston extends,the OBJECT of including an EFFECTIVE quench area is to force a jet of rapidly moving fuel/air mix to shoot out into the combustion chamber, resulting in a far faster and more efficient burn in the combustion chamber, clearance of less than about .036 results in the piston hitting the heads in some applications, distances over about .044 results in a far less effective jet of fuel/air mix and potential for detonation to occur in the quench area, remember it only works if the head and piston get so close that theres a boundary layer of semi cooled air that's so tight it can,t ignite.
quench acts a bit like throwing a cup of gasoline, hard and fast into a camp fire.......it results in a huge increase in the burn rate compared to pouring the gas slowly into a fire
keep in mind ONLY pressure resulting from the fuel/air mix occurring AFTER TDC adds power or torque, any pressure or fuel burnt during the compression stroke tends to reduce power
example
if the piston was .020 down the bore at TDC and the compressed head gasket was .024 the quench distance would be .044, but in reality at high rpms its a bit tighter because pistons and rods stretch at high rpm levels just a bit.
try to keep the QUENCH in the .038-.042 for the most effective results, naturally milling the heads has no effect on quench, but milling the block does, simply because while milling the heads raises compression, by making the combustion chamber smaller in most engines it doesn,t change the quench distance, milling or decking the block changes the distance between the piston and head surface so that does alter quench.
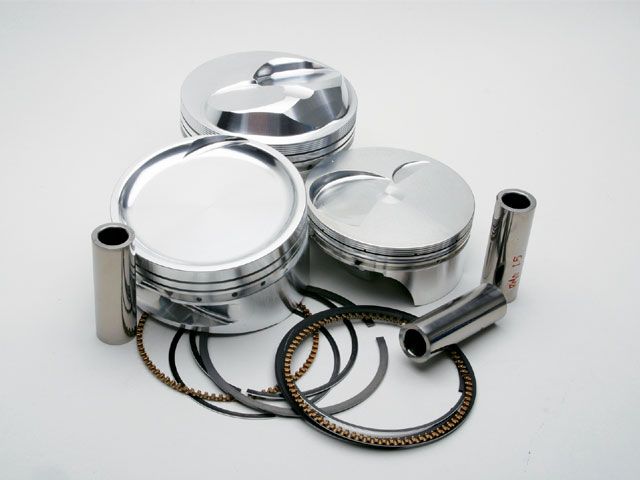
SQUISH
An area in the combustion chamber of some engines where the piston squishes or squeezes part of the fuel-air mixture at the end of the compression stroke. As the piston approaches top dead center, the mixture is pushed out of the squish area and this promotes turbulence, further mixing of the fuel-air mixture and more efficient combustion
run less than about .037 thousands and at high rpm levels the pistons might hit the cylinder heads, run more than about .044 thousands the QUENCH effect of forcing the fuel air mix to the center of the cylinder from the cylinders edge area looses both speed and effectiveness, remember the quench area must be so tight that virtually all the fuel/air mix is forced (squished) into the center area and none is allowed to burn until its squirted into the burn area increasing turbulence and burn efficiency
in theory the much better quench, combined with the shorter more compact area the flame front needs to cover and the far higher turbulence combine to allow more of the pressure to build AFTER the crank passes TDC on the end of compression and beginning of the power stroke
its mostly an advantage in that you get a more even and FASTER burn in the cylinder and less chance of detonation, simply because both the lower time and faster pressure curves favor the ignition flame front vs detonation
look, it takes approximately 40 thousands of a second for the flame from the ignition to cross a 4.25" bore,at low rpms and still takes about 15 milliseconds at high RPM due to the much faster movement of the compressed fuel air mix in the cylinders, lets look at what that means
if the Chevy plug is located 4/5ths of the way to one side that's a time of about 32 thousands for the pressure to build as the flame travels 3.4" in the Chevy but in a compact combustion chamber it could only take the cylinder flame front less than 10-20 thousands of a second to travel across the combustion chamber for a complete burn at low rpms, this of course speeds up as the swirl and turbulence increase with increased engine RPMs but the ratios stay similar. this results in more usable energy WORKING on the piston AFTER IT PASSES TOP DEAD CENTER ON THE POWER STROKE. BUT MODERN WEDGE combustion chambers use increased QUENCH to speed the flame front and lower the burn time combined with a smaller combustion chambers.
the difference may be easier to grasp if you think of the quench area as a significant part of the total combustion chamber volume,that's forcing its potential fuel/air mix into the central combustion chamber as a jet of highly compressed F/A mix, like the difference between lighting a cup of gasoline by simply placing it next to a camp fire vs throwing it violently into a camp fire
naturally you need to verify the piston to deck height

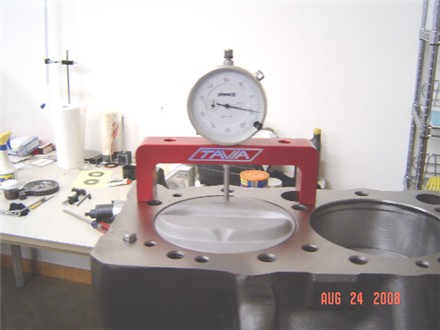
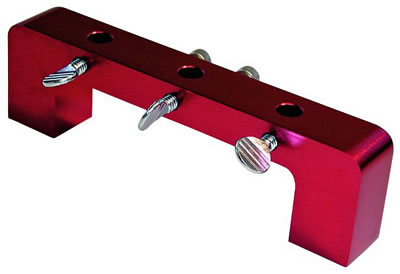
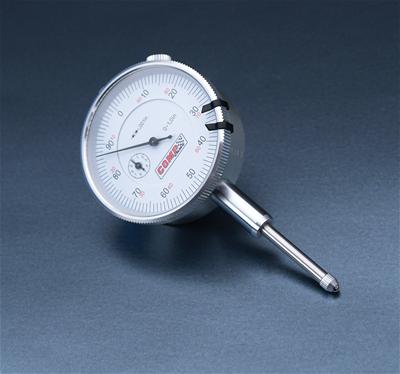
the quench (squish) at .035 would be unlikely to be a problem from the octane being to low, angle, but rather a huge potential for the piston too head contact as the rpms build and the rods stretch on the exhaust strokes where there's little compression to slow the rod stretch as they play (crack the whip) at high rpms and if you don,t think rods and pistons expand under high rpm and high heat then you've probably never seen the marks the heads quench areas leaves on heads/pistons at quench distances lower than about .037 which Ive found its best to exceed and stay in the .038-.042 range.
yes its entirely possible to run a .035 quench, but you better be running top quality components and measure very carefully.
get out a .035 feeler gauge and look at it on edge then rock the piston in the bore slightly and you think about it a bit.
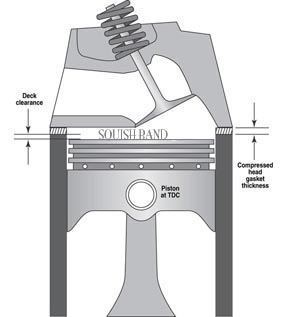
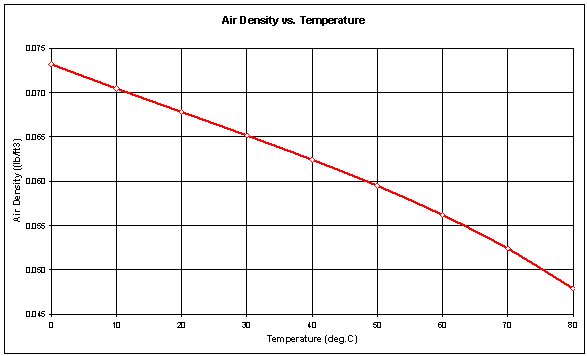
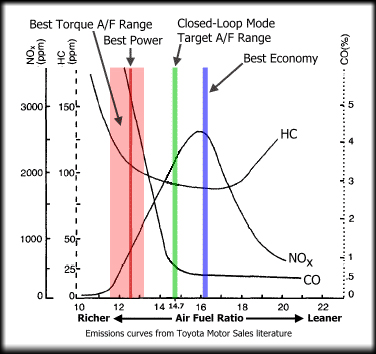
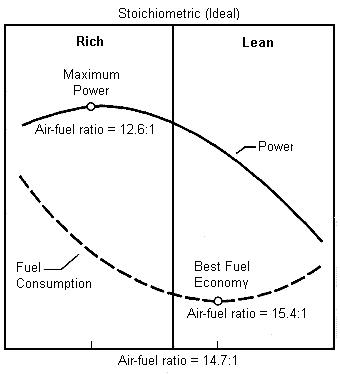
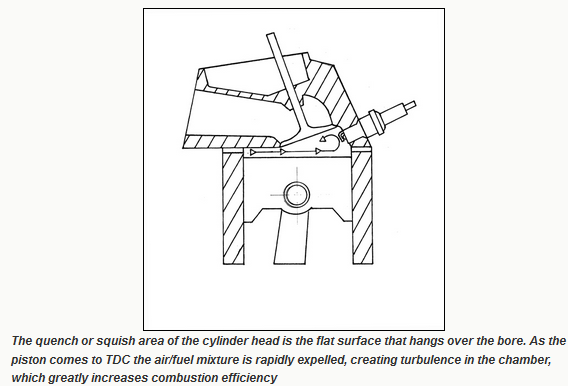
http://em-ntserver.unl.edu/Mechanics-Pages/Luke-schreier/unzip/Tension and Compression in Connecting Rods VI.htm
the quench/squish area is generally located opposite the spark plug location side of the bore, and there's generally a matching flat area on the combustion chamber
here the dome and spark plug side is on the right and the flat quench/squish is on the left


here is a cylinder head

the spark plug and dome goes to the exhaust side,(lower edge) the quench squish area is the matching flat area towards the intake on these sbc heads (upper edge in this picture)
the idea, is that as the piston almost impacts the head the two flat areas force the cylinder volume trapped between the flat areas violently toward the spark plug , this speeds the burn rate and tends to limit detonation
think of clapping your hands together violently with a raw egg in your palm, that raw egg will be forced away from the impending impact and squeezed out to spray to the sides, the cylinders volume get sprayed the same way but limited by the bore and chamber in only towards the spark plug direction, thus a high velocity mist of gas and air gets thrown into the area of ignition just as the burn starts, speeding the burn rate and lowering the distance the flame must cross
look at this picture, in a real engine the distance is very tight unlike the diagram and a good percentage of the cylinder volume is compressed and forced into the dome/spark plug area
generally you measure the piston to block/deck height and then add the compressed head gasket thickness to the deck height distance to find the heads distance from the piston, but cross check with
a dial indicator on a deck bridge
http://store.summitracing.com/partdetail.asp?autofilter=1&part=PRO-66797&N=700+115&autoview=sku
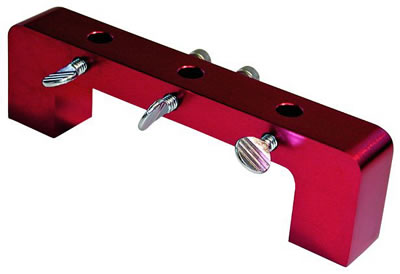
and a dial indicator
http://store.summitracing.com/partdetail.asp?autofilter=1&part=PRO-66962&N=700+-111345+115&autoview=sku
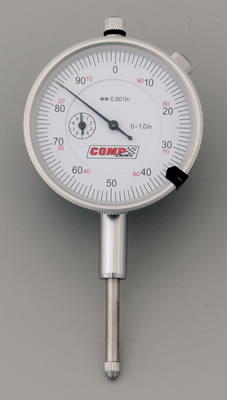
and re- check with plasti-Gage during the pre- assembly
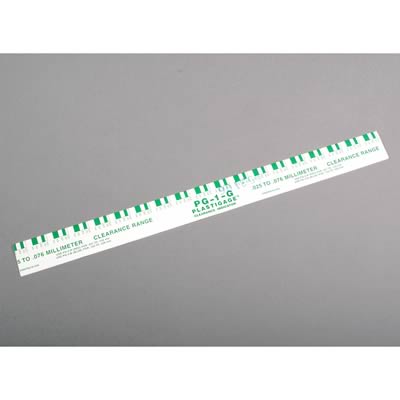
http://www.kb-silvolite.com/article.php ... ad&A_id=56
http://www.kb-silvolite.com/article.php ... ad&A_id=36
http://www.cswnet.com/~carother/compression_ratio.htm
http://www.kb-silvolite.com/test/articl ... ad&A_id=35
and before someone points out that plasti-Gage won,t measure .038-.042, yeah! your correct by itself it wont but Ive cut dime size end tabs from a cheap feeler gauge
http://www.mytoolstore.com/kd/kdfeel01.html

next time your looking
ASK FOR A TAPPET GAUGE
for some reason most auto parts stores don,t seem to have feeler gauges but do have (TAPPET GAUGES)
http://www.jcwhitney.com/jcwhitney/prod ... map=27994G

and I usually use a .035 tab with a cross of plasti-gauge on top set on the quench area, its easily placed and removed and measured after spinning the engine over and there's no chance of damage to the piston or head,
lots of guys just use soft solder and a machinists mic., and it works fine in most cases, the potential problem with that is that solder forces the piston to compress the solder and it takes a good deal MORE force to compress solder than a thin thread of plastic, when the rods in compression you'll get a similar clearance, but when the rods whipping the piston around on the exhaust stroke under TENSION that quench distance tends to be closer to the head as the rod,piston and clearances tend to stretch out due to heat and inertial loads due to centrifugal forces, you need to be sure the piston wont hit the head on both the exhaust and compression strokes and holding the piston down, away from the head crushing solder tends to give a slightly wider clearance because it tends to rock the piston in its bore, firmly away from the quench clearance on its piston pin.
yeah! Ive done it both ways and the difference is minor but there is still a difference of a couple thousands, probably not significant in most cases but get that clearance real tight and it might be critical info to know
ID suggest trying for a MINIMUM of .038-.044 on a 496 BBC for quench as the long stroke bbc, or any BBC run over about 6000rpm with a 4" or longer stroke) tends to allow the quench clearance distance to tighten up a bit at high rpms, as parts stretch under stress/inertial loads
Last edited by a moderator: